
Research in Zhang Jun's group
Laser Cooling in Condensed Matter
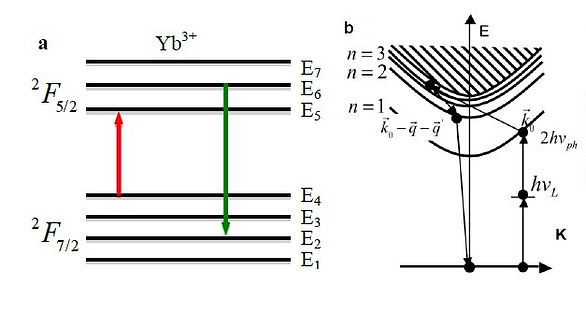
Optical irradiation accompanied by spontaneous anti-Stokes emission can lead to cooling of matter, a phenomenon known as laser cooling or optical refrigeration proposed in 1929 by the German Physicist Peter Pringsheim[1]. In gaseous matter, an extremely low temperature in dilute atomic gases can be obtained by Doppler cooling[2], in which the translation energy of atoms is removed by the spontaneous emission followed by the absorption of the detuned laser beam. In solid state materials, laser cooling is achieved by the annihilation of phonons, quanta of lattice vibrations, during anti-Stokes luminescence, hence solid state materials have no translational moment and energy. Since the first experimental demonstration in rare-earth (RE) doped glasses[3], considerable progress has been made particularly in ytterbium-doped glasses or crystals with a recent record of ~110 Kelvin cooling from ambient[4], and 40 Kelvin net laser cooling in II-VI semiconductor CdS that harnessed by mutiphonon assisted anti-Stokes resonance and strong electron-phonon coupling[5]. Our researches focus on understanding the physical mechanisms of laser cooling in semiconductors, finding the possibility of laser cooling below 77 Kelvin, and building an all-solid optical cryocooler.
References
[1] Pringsheim, P. Zwei Bemerkungen über den Unterschied von Lumineszenz- und Temperaturstrahlung. Z. Phys. A 57, 739-746 (1929).
[2] Chu, S., Cohen-Tannoudji, C. & Philips, W. D. For development of methods to cool and trap atoms with laser light. Nobel Prize in Physics, http://nobelprize.org (1997).
[3] Epstein, R. I. et al. Observation of laser-induced fluorescent cooling of solid. Nature 377, 500-503 (1995).
[4] Seletskiy, D. V. et al. Laser cooling of solids to cryogenic temperatures. Nature Photon. 4, 161-164 (2010).
[5] Zhang, J., Li, D., Chen, R. & Xiong, Q. Laser cooling of a semiconductor by 40 Kelvin. Nature 493, 504 (2013).
Phonon Based Quantum Control in Solids

Spontaneous Raman and Brillouin scattering is described in terms of the statistical nature of the fluctuations in the optical properties of the medium. Incident photons are inelastically scattered to a lower (Stokes) or higher (anti-Stokes) frequency via electron-phonon interactions, which correspond to the creation or annihilation of phonons, respectively. When the scattered photon frequency resonates with an internal state (such as electronic transitions), the corresponding frequency of the phonon is larger than the damping of the internal state, and the corresponding scattering peak of the phonon exhibits the highest intensity. Depending on the resonant situation, the energy-flow direction of scattering can be controlled either as phonon heating pumped at a blue-resolved-sideband pumping (Stokes resonance) or phonon cooling at a red-resolved-sideband pumping (anti-Stokes resonance). The highest cooling or heating efficiency is achievable at the first resolved sideband, that is n = 1. Such a technique is called resolved-sideband cooling, which has been demonstrated at low-frequency oscillators in trapped atoms and cavity optomechanical systems. Resolved-sideband cooling plays an important role in the preparation and coherent manipulation of pure quantum states, such as the generation of Fock, coherent and squeezed states, and of Schrödinger cat states, and quantum logic gates, which represents the first step toward quantum computation.
The two main barriers for creating superpositions and entanglement in the mechanical motion of macroscopic systems are strong internal interactions, which complicate the dynamics, and strong coupling with the environment, which leads to short decoherence times. The standard approach is to design systems with well-defined and long-lived normal modes that can be selectively excited, and then to cool them to remove thermal noise and isolate them from the environment. Substantial progress has been made toward demonstrating strong quantum signatures in larger systems-for example, in optomechanical, molecular, and superconducting systems. A different approach is required, however, to reveal quantum features in the motion of “ordinary” solids in the high-entropy environment present at ambient conditions. Without a specially engineered system, measurements must be made on time scales shorter than the characteristically fast coherence decay times of a real-world system.
Our group not only focuses on acoustic phonon of optomechanical systems, but also the motion of “ordinary” solids such as optical phonon (vibrational) modes of bulk crystal to study quantum physics and quantum control, ultrasensitive sensing.
References
[1] Nature Photonics, 6, 41 (2011). [2] Science, 334, 1253 (2011). [3] PRL 101, 073002 (2008).
[4] K. F. Reim, J. Nunn, V. O. Lorenz, B. J. Sussman, K. C. Lee, N. K. Langford, D. Jaksch & I. A. Walmsley, Nature Photonics 4, 218 - 221 (2010).
[5] Markus, A., Tobias J., K. & Florian, M. Cavity optomechanics. Rev. Mod. Phys. 86, 1391-1452 (2014).
[6] J. Zhang, L. C. Kwek, and Q. H. Xiong, Resolved sideband Raman cooling of an optical phonon in semiconductor materials, Nature Photonics, DOI: 10.1038/nphoton.2016.122 (2016).
Optical Physics of Low Dimensional Quantum Materials
References
[1] X. F. Qiao, J. B. Wu, L. W. Zhou, J. S. Qiao, W. Shi, T. Chen, X. Zhang, J. Zhang, W. Ji, P. H. Tan, Polytypism and Unexpected Strong Interlayer Coupling of two-Dimensional Layered ReS2, Nanoscale, 9, 8324-8332 (2016).
[2] X. Lu, M. Utama, J. H. Lin, X. Luo, Y. Y. Zhao, J. Zhang, S. T Pantelides, W. Zhou, S. Y. Quek, Q. H. Xiong, Rapid and Nondestructive Identification of Polytypism and Stacking Sequences in Few‐Layer Molybdenum Diselenide by Raman Spectroscopy, Adv. Materials, 27 (30), 4502-4508 (2015).
[3] J. Zhang, C. Cao, X. L. Xu, C. H. Liow, S. Z. Li, P. H. Tan, and Q. H. Xiong, Tailoring plasmonic metamaterials in optical frequency: coupling, dispersion and sensing, ACS Nano, 8, 3796-3806 (2014).
[4] Y. Y. Zhao, X. Luo, H. Li, J.Zhang, P. T Araujo, C. K. Gan, J. Wu, H. Zhang, S. Y.Quek, M. S Dresselhaus, Q. H. Xiong, Interlayer breathing and shear modes in few-trilayer MoS2 and WSe2,Nano Lett., 13(3),1007-1015 (2013).
[5] Q. Zhang, X. Liu, M. I. B. Utama, J. Zhang, M. de la Mata, J. Arbiol, Y. H. Lu, T.C. Sum and Q. H. Xiong, Highly enhanced exciton recombination rate by strong electron-phonon coupling in single ZnTe nanobelt, Nano Lett., 12(12), 6420-6427 (2012).
[6] D. H. Li, J. Zhang, Q. Zhang, and Q. H. Xiong, Electric-field-dependent photoconductivity in CdS nanowires and nanobelts: exciton ionization, Franz−Keldysh, and Stark effects, Nano Lett., 12(6), 2293-2999 (2012).
[7] Q. Zhang, J. Zhang, M. I. B. Utama, B. Peng, M. de la Mata, J. Arbiol, and Q. H. Xiong, Exciton-phonon coupling in individual ZnTe nanorods studied by resonantRaman spectroscopy, Phys. Rev. B, 85(8), 085418 (2012).
[8] J. Zhang, Z. P. Peng, A. Soni, Y. Y. Zhao, Y. Xiong, B. Peng, J. B. Wang, M. S. Dresselhaus, and Q.H. Xiong, Raman spectroscopy of few-quintuple layer topological insulator Bi2Se3 nanoplatelets, Nano Lett., 11(6), 2407-2414 (2011).
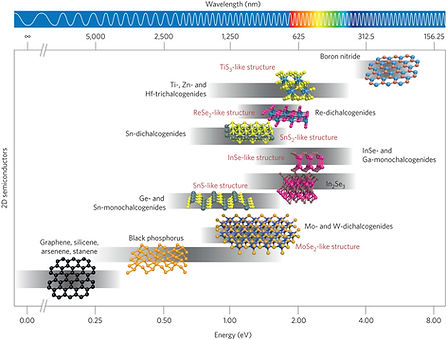
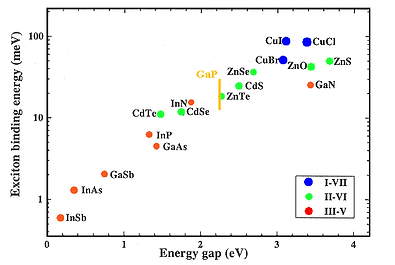